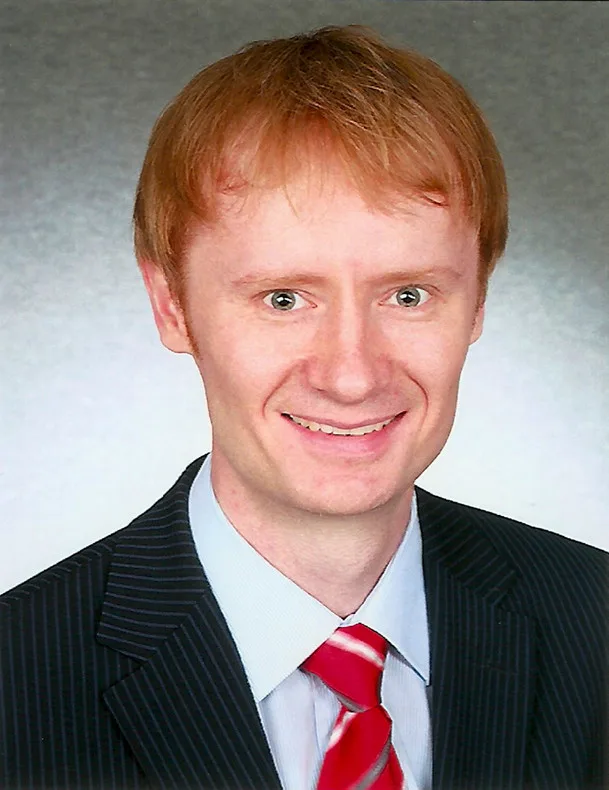
Meet Dr. Alex Greilich, an experimental physicist who started his journey from Russia to his current endeavors in Germany.
Venturing beyond mere theory, he dives headfirst into the intricate world of semiconductor nanostructures and spin dynamics, unearthing remarkable phenomena like time crystals along the way. Collaborating with esteemed institutions such as the Ioffe Institute, (research center within the Russian Academy of Sciences) Dr. Greilich’s work pushes the boundaries of what we know about the universe.
His expertise shines brightest in the realm of coherent optical control of spin states, where he skillfully employs laser pulses to manipulate with precision. Through innovative techniques like spin noise spectroscopy, he unravels the enigmatic mysteries surrounding spin dynamics, paving the way for groundbreaking advancements.
When he’s not immersed in the complexities of quantum exploration, you can find him embracing the great outdoors through activities like running and rock climbing.
For those inspired by his journey, Dr. Greilich offers invaluable advice, emphasizing the importance of collaboration, resilience, and a profound understanding of the subject matter.
Embark on an enthralling adventure into the world of experimental physics alongside Dr. Alex Greilich – you won’t want to miss it!
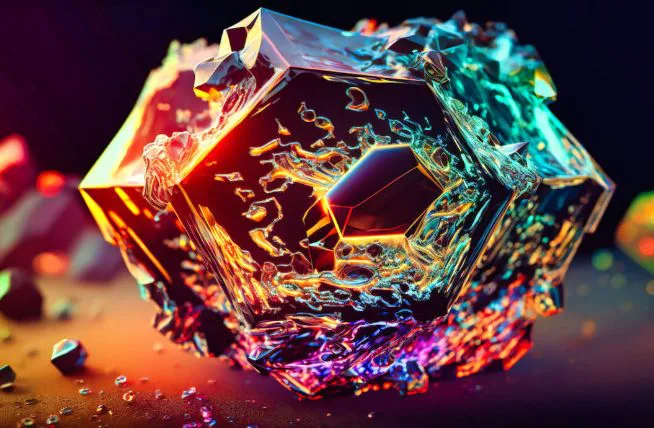
Can you share a bit about your background and what inspired you to pursue a career in experimental physics?
I’ve started my physics education in Russia, and did my bachelor’s in physics. I was theoretically working out how to describe the pulsation of glaring meteors when this go through the atmosphere. It was fun time. I was learning how to work analytically through the equations and learned the basics in numerical methods using PCs.
My master’s degree in physics I was doing in Germany. It took me some time to learn German when I moved here, and my initial thought was to go into high energy physics and study elementary particles, but after listening to the course on Environmental and Bio Physics I decided to stay in mesoscopic area. So, I did my thesis again in theory, on chaos-physics. I was figuring out how to make a chaotic system predictable by implementing certain pinning points into it. This time, I’ve learned a lot about numerical methods and programming.
I started to think about PhD, as science was bringing me a lot of joy. At the same time, I’ve had enough of sitting and breaking my head on the abstract numerical problems. Once, walking home, I met by a chance one of professors whose courses I was visiting while I was a student. He was already retired, but sad that there was a new professor setting out new labs who is also searching for new students. So, I decided to try it out and in one moment become an experimentalist. Until now, I don’t regret this decision. I really enjoy working in the lab.
I’m particularly curious to know more about your recent research on time crystals, what led to the choice of indium gallium arsenide for designing the crystal? And how does the interaction between nuclear spins and electron spins contribute to the formation of the time crystal?
Well, it’s all started as a side-project, a curiosity driven research. I’ve read about Geim and Novoselov, that they like to try out some things in the lab, which are not related to their current research, like levitating the frog in a high-magnetic field, or develop a surface structure, which is sticky like gecko-skin, and so on. I found it quite amusing and decided to do it also.
Out department had a very tight connections to the Ioffe Institute, in Saint Petersburg, Russia. So, I met a lot of bright scientists and was talking about different ideas. At some moment, the idea came up, if one can find a chaotic behavior in electron-nuclear spin system. As I was already familiar with chaos from my master times, I found it very appealing to try. So, we started to work on it.
First, we have reproduced the structure with AlGaAs, that showed the auto-oscillations. It was known to show it since the work of Novikov and Fleisher from 1978. Unfortunately, it was very slow, there was only one period in 10 minutes. I was already doing a lot of research on InGaAs material at that moment, so it was an obvious material to try. We have found the chaotic behavior we were looking for, and realized, that it’s having much bigger parameter areas of periodicity, which, as we have realized, satisfy the time-crystal conditions.
To the second question: The theory of the oscillation was also worked out by Soviet scientists, D’yakonov, Merkulov and Perel’, in 1980. In a simple way one can see the oscillations as a never-ending catch-up game. Using the laser excitation, we polarize electron spins (S). These polarize the surrounding nuclear spins using the hyperfine interaction (BN). If the structure, in this case InGaAs, has a strain in it, which is distributed in different directions, it leads to orientation of nuclei quadrupole moments along the strain, which causes an effective quadrupole nuclear polarization (BQ), once nuclei become polarized. So, after the polarization of nuclei, their polarization turns into the direction of the quadrupole induced fields. This reorientation reduces the electron spin polarization, which in its turn reduces the quadrupole induced nuclear spin polarization. So, it closes the loop of a cycle. I repeat it in symbols: S creates BN:
[BN changes due to BQ -> S changes due to BN -> BQ changes due to S ]X
This is oscillating with the time proportional to the nuclear spin relaxation.
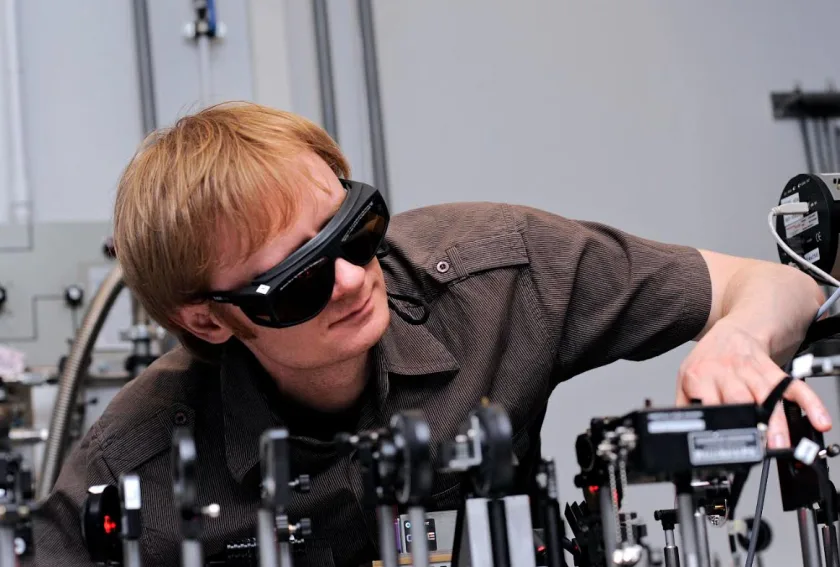
Can you elaborate on how your group achieves coherent optical control of spin states in semiconductor nanostructures?
For the coherent control one uses the laser pulses. These serve in several ways:
- The laser pulse creates electron-hole pair, ones applied at the resonance to the exciton/trion transition in a semiconductor. Depending on the laser polarization, one can define the spin polarization of electron and hole, due to selection rules and band structure of the semiconductor.
- If the polarized carrier spin is already defined by a previous illumination, one can use additional laser pulses to manipulate the spin orientation. This is done by using the Stark effect. Ones the control laser is spectrally detuned from the optical transition, its electrical field component creates an effective magnetic field along the light propagation, which is proportional to the laser pulse area (this is defined by the laser pulse duration and amplitude) and inversely proportional to the laser detuning from the optical transition. It allows one to coherently rotate the spin on the picosecond timescales at different angles.
Could you provide insights into the non-perturbative spectroscopy techniques your group employs? What advantages do these techniques offer in comparison to traditional spectroscopy methods?
Yes, we use the spin noise spectroscopy as a nearly non-perturbative method. At the heart of this methos lies the dissipation-fluctuation-theorem. Which tells you basically, that one can retrieve the same information from the system, by either observing how it decays after an excitation (dissipation of energy) or by observation of systems fluctuations without excitation. For this we also use optical methods, developed by two soviet scientists, Alexandrov and Zapasskii, in 1981.
We test the system in its ground state using the linearly polarized laser light. Once it is transmitted through the semiconductor, we map the magnetization fluctuations (fluctuations of spins) onto the light polarization by Faraday effect, and finally measure the rotation angle of the polarization plane.
Using fast diodes, ADC and FPGAs we do a fast Fourier transform to measure the spectra of the fluctuations. From it one can determine the carrier spin relaxation time, Lande g-factors and so on. This method allows to extract some additional information, which one cannot have by using the linear type of spectroscopy, like absorption, transmission, or a Faraday rotation.
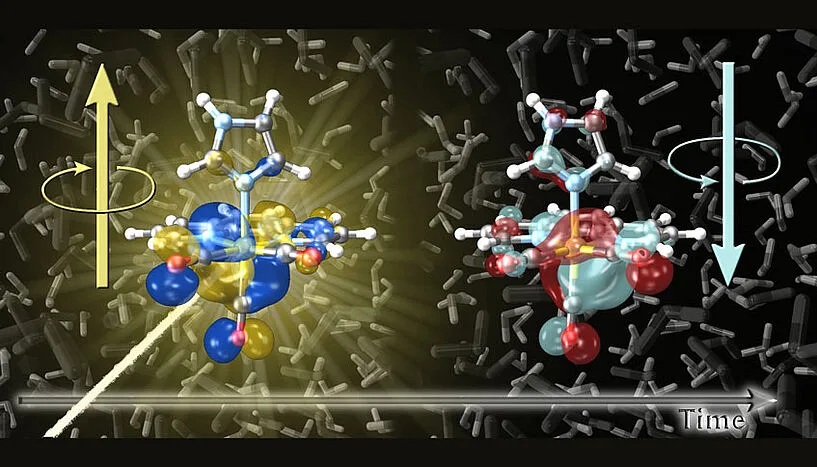
Could you explain the concept of long-range spin-spin entanglement and how it is achieved using intrinsic coupling to photons?
This is based on the idea of spin-photon entanglement. Ones the single excited state recombines by sending out a photon and leaving a single carrier spin behind, these two can be entangled.
If we combine two indistinguishable photons, both entangled with its own spin, on a beam splitter and measure them as a single click (where both come to the same detector) we entangle the two remote carrier spins. This is called the “measurement induced entanglement” scheme.
Where do you see the future directions of your research heading, and are there specific goals or milestones your group is aiming to achieve?
Now, as we have realized the time crystal, it would be nice to find some practical application for it. Furthermore, there are some interesting ideas in terms of spin-noise spectroscopy. It would be interesting to use it to study some magnetic materials, while they undergo the phase transitions. These are the conditions with a lot of fluctuations.
What are your other interests besides academic commitments and research responsibilities… reading, painting, gardening, skiing maybe?
I enjoy reading, running, sailing, diving, and rock climbing. I kind of combine different types so activities, scientists like to do.
Someone comes up to you and says, “I wanna be just like you. I want to be an Experimental Physicist”. What advice would you give?
“Run!” If this doesn’t help, I’ll say: “Eliminate your competitors by making them your collaborators. And, you need to be robust to frustrations and understand what all of your devices and programs are doing at all time.”
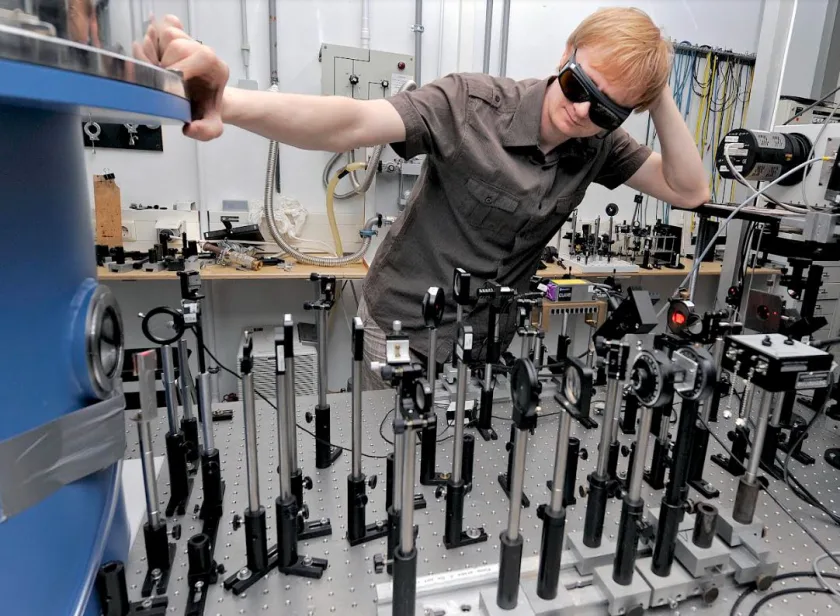
Quick bits:
What is your favourite movie quote?
“Ku!” – Kin-Dza-Dza!
If you were a superhero what would your powers be?
I would like to be able to zoom in/out of space and slow down/accelerate the time to see the processes on different time and space scales.
It would be also interesting to immediately know why something is not working the way it should.
What would you do on Mars for fun?
I would break the Earth record for high jump.
What will your TED Talk be 10 years from now?
How you should “prepare the stage” for a luck to happen in science.
What books should I read in 2024?
“Human kind” by Rutger Bregman
(Wow! Thank you, Dr. Greilich, for an incredibly inspiring conversation! Your work is a true source of inspiration. We eagerly anticipate our next visit to witness more of your innovative research. Until then, we extend our best wishes for your continued success in all your future endeavors.)