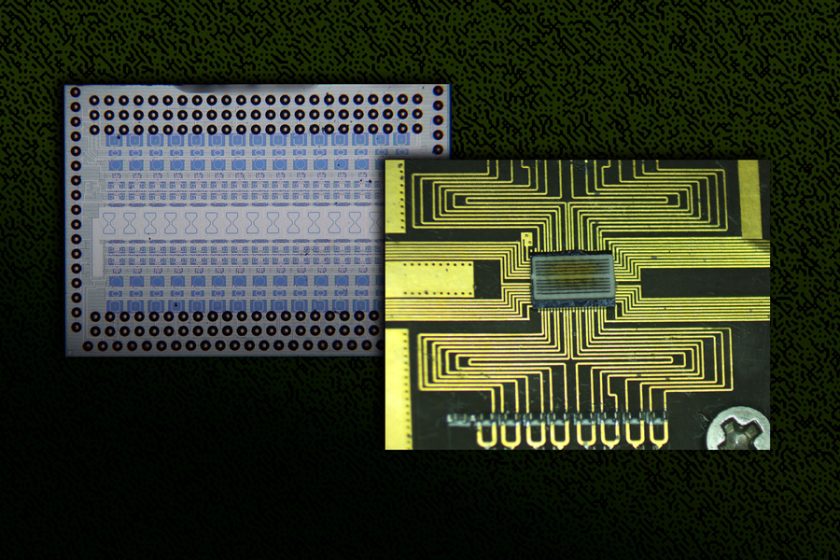
Researchers at MIT have built a small chip that can generate terahertz waves more effectively and more affordably than what’s currently out there. That’s huge, ‘cause terahertz waves sit between radio and infrared on the electromagnetic spectrum, so they can carry more data than radio waves and see through more materials than infrared light. This makes them a promising candidate for faster wireless communication, sharper medical imaging, better security scanners, and smarter environmental monitoring.
Why Most Terahertz Signals Get Stuck in the Chip
The problem is, making terahertz waves on a silicon chip isn’t easy. Normally, when these waves hit the back of a chip, most of them bounce back instead of passing through. That’s because silicon has a high dielectric constant. The dielectric constant is like a property of a material that affects how electromagnetic waves talk to or move through it. Here, it reflects too much.
Some systems try to fix this with large, expensive silicon lenses that help the waves escape. Silicon lenses help boost terahertz wave transmission by focusing and directing the weak signal that manages to escape the chip. But those lenses are bulky and don’t scale well if you’re trying to build a whole array of chips.
A Simpler Fix That Lets the Signal Out
The MIT team found a cleaner solution. They added a thin sheet to the back of the chip, something cheap and commercially available. And then used a laser to poke tiny holes in it. These holes act like tiny pockets of air, lowering the sheet’s dielectric constant so it matches better with both the silicon and the surrounding air. That lets more of the terahertz signal pass through instead of reflecting back into the chip.
On top of that, they used custom transistors from Intel that can handle higher frequencies and voltages than typical chip components. So the chip puts out a stronger signal without needing the bulky lens setup.
The results were solid. The chip reached a peak radiation power of 11.1 decibel-milliwatts, which beats other similar systems. And because it’s built using standard chip-making processes, it’s a lot easier to scale up.
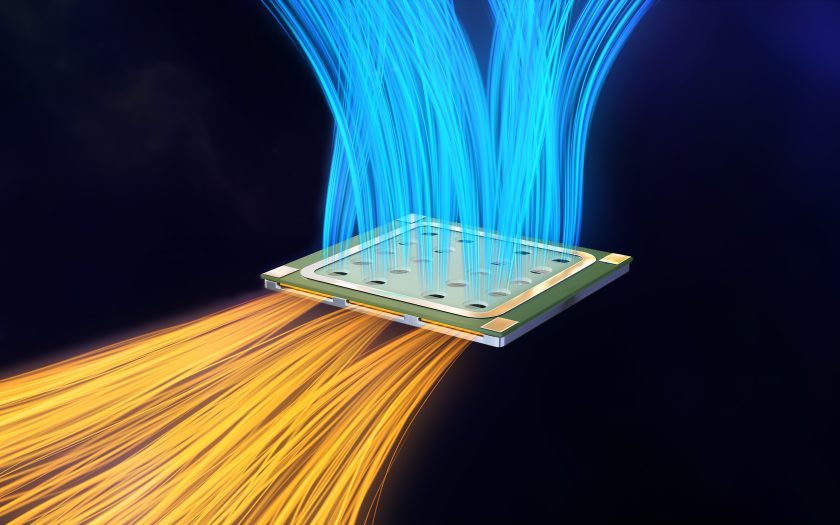
Steering the Beam Without Moving a Thing
Getting to this point wasn’t that straightforward either. Terahertz generation involves high power and heat, so the team had to move beyond the usual chip design playbook. They also had to figure out how to attach the matching sheet in a way that works for large-scale manufacturing.
Next, they’re aiming to build a phased array of these chips, which could steer and focus the beam, kind of like how satellite dishes work, but in a much smaller form.
A phased array is basically a bunch of tiny antennas (or in this case, chip-based terahertz sources) working together to send or receive signals in a specific direction, without actually moving anything.
Instead of pointing a dish or antenna at your target, a phased array tweaks the timing (or phase) of the signals from each tiny part. If you do it right, all the signals line up in one direction and cancel out in the others. This tricks the beam into pointing where you want electronically, just by tweaking the phase delays, no moving parts or motors needed.
It’s like a bunch of people clapping together, if everyone claps at the same, the sound is loud and focused. But if they clap a little off, the noise gets messy or even cancels out. A phased array just tunes the “clap timing” of each chip so it points the energy right where it’s supposed to go.
Takeaway
Using a thin, patterned sheet and powerful transistors, MIT has opened the door to low-cost chips that are easier to make and much easier to integrate into devices we already use. A few possible uses:
- Faster wireless data: it’ll be about ultra-fast streaming or downloads
- Sharper medical imaging: something like MRI-level detail without harmful radiation
- Smarter security scanners: that can spot hidden objects more clearly
- High-res radar: for drones, vehicles, or satellites
- Air quality and pollution tracking
- Chemical detection and industrial quality control
Eventually, this could make electronics way better and more sensitive, working in the terahertz range, something we haven’t really nailed on a big scale before.