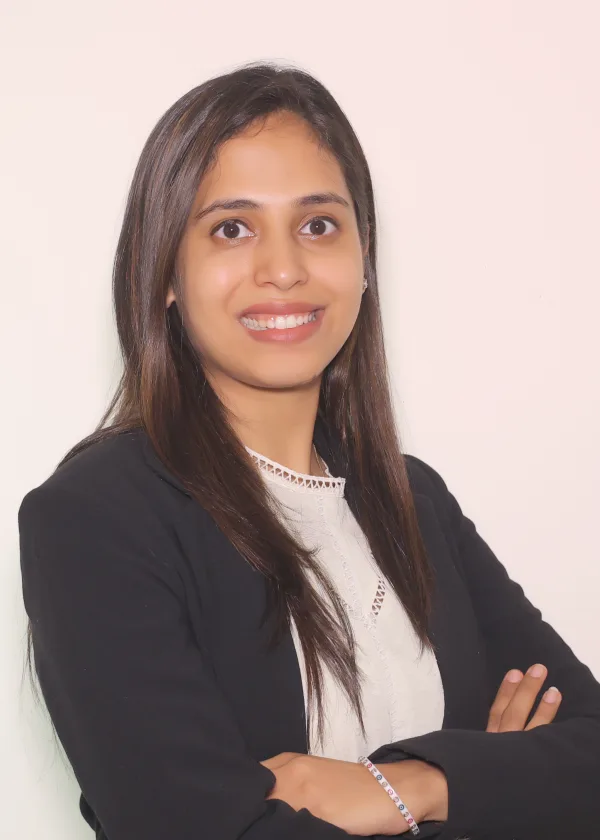
In a rapidly evolving world where technological advancements meet the growing demand for sustainability, Dr. Sukriti Hans stands at the forefront of materials research. Currently a Junior R&T Associate at the Luxembourg Institute of Science and Technology (LIST), Dr. Hans is playing a pivotal role in developing high-resolution characterization methods that can redefine the future of materials science.
As part of an EU-funded initiative, her work focuses on leveraging advanced techniques like FIB-SIMS to analyze breakthrough materials, with applications spanning the automotive, textile, and cosmetics industries. With a passion for sustainable innovation, she is contributing to a greener, smarter future through her research and dedication. Despite her impressive achievements, she stays humble, approaching every challenge as a fresh opportunity to learn – much like unlocking new levels in a game.
Join me as we explore Dr. Hans’s remarkable journey fueled by curiosity, growth, and a passion for scientific discovery!
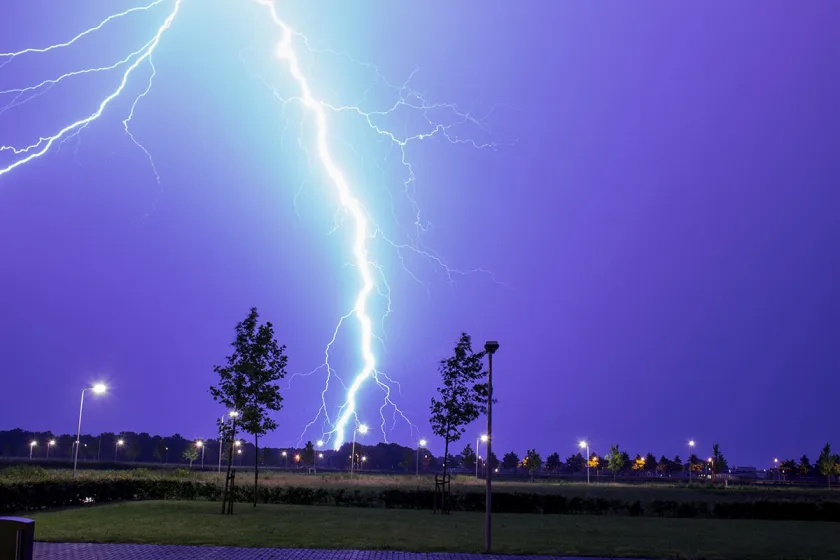
How did you get into Plasma Physics and Ion Beam Nanopattern Formation, and what motivated you to pursue it as a career?
My interest in Plasma Physics really took shape during my master’s studies at Delhi University, where I first encountered the subject. While studying plasma physics, I had the opportunity to intern at the Institute for Plasma Research (IPR). There, I worked on the Aditya-U tokamak, where I studied the spectral emissions from impurity ions within the plasma. By monitoring the UV spectral lines of C4+ (He-like) and C2+ (Be-like) ions, we could investigate carbon transport and plasma edge rotation, which are vital for enhancing plasma confinement and performance.
This experience sparked my fascination with plasma physics and its numerous applications. After realizing the broad potential of plasma technologies, I decided to pursue my PhD in the field, and from there, my focus expanded into ion beam nanopattern formation, where I saw exciting possibilities in nanotechnology.
You’ve consistently demonstrated academic excellence throughout your undergraduate studies, earning multiple awards and top rankings. What strategies or habits did you develop during your time at university that you believe were key to maintaining such high academic performance year after year?
Honestly, it was less about rigid strategies and more about staying curious and engaged with the subjects I was passionate about. I didn’t aim for perfect grades, but rather tried to understand the core concepts deeply. I did develop a habit of reviewing notes regularly, which helped me avoid last-minute cramming.
Also, having a study routine that wasn’t overwhelming but consistent made a difference. If I ever struggled with a topic, I wasn’t afraid to ask for help or collaborate with friends. Balancing studies with hobbies and spending time with family helped me recharge, keeping stress in check and making the learning process more enjoyable.
Talking about your current research, can you explain in simple terms what ion beam sputtering (IBS) is and how it creates patterns like nanoripples and terraces on glass surfaces?
Ion beam sputtering (IBS) involves bombarding a material’s surface with energetic ions, causing atoms to be ejected and forming nanoscale patterns like ripples and terraces. In my work with soda-lime glass, I used low-energy ions to precisely modify the surface. These energetic ions create patterns without causing deep damage to the material, making it a controlled and effective method for tuning surface properties like wettability.
The ability to form these patterns is especially useful for applications like making glass surfaces hydrophobic, improving adhesion in thin films, or enhancing optical properties, while maintaining the material’s structural integrity. By carefully adjusting parameters like ion energy, angle, and fluence, we can create durable and functional nanopatterns for technologies like solar panels, sensors, and bio-sensing devices.
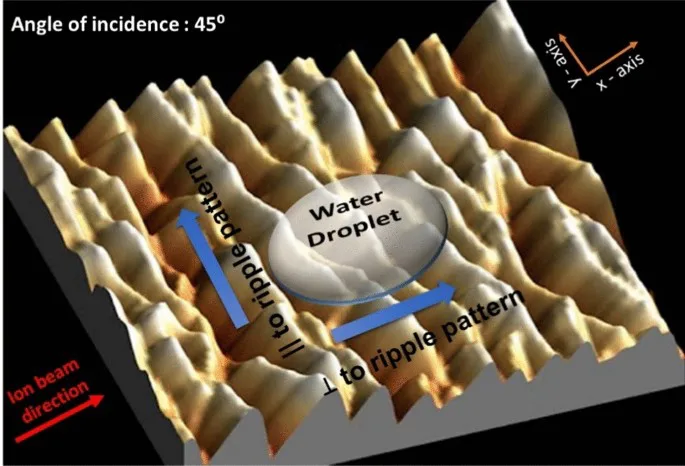
In your study, you mention the transition from ripple patterns to terrace formations. Could you explain what causes this shift and why it’s important for your research?
The transition from ripple patterns to terrace formations during ion beam sputtering (IBS) is influenced by several factors, including ion energy, angle of incidence, and ion fluence. As ion energy increases, the surface undergoes a significant transformation from sinusoidal ripple patterns to more uniform terrace-like structures. This change is driven by the surface dynamics that favour a stable, low-energy state.
Our experimental observations show that at lower ion energies, the surface develops sinusoidal ripple patterns. As the energy increases, the surface evolves into terraces. This shift occurs because higher ion energies cause more significant surface disruptions, leading to the formation of terraces as the surface seeks a more stable configuration. This is consistent with continuum equations and theoretical models that predict such behaviour, indicating that the transition is linked to cubic nonlinearity in the surface evolution process.
The formation of terraces rather than ripples is crucial for applications where surface stability and controlled wettability are important. For instance, in creating hydrophobic surfaces for optical and sensor technologies, having a stable terraced structure can enhance performance and durability without compromising transparency. Our findings demonstrate that by tuning ion beam parameters, we can achieve desired surface properties effectively, which is valuable for various industrial applications.
Your study uses Xe ion irradiation at 0.5 keV. What made you choose this specific ion and energy level, and how does it impact the surface of silicon compared to other ions or energies?
I chose Xe ions at 0.5 keV for several reasons. Initially, I aimed to study the triangular features observed with lower energy Ar ions (around 500 eV). However, these patterns were subtle with Ar ions, prompting a shift to Xe ions, which are heavier and provide different interaction dynamics. At 0.5 keV, Xe ions struck a balance between sufficient energy to induce notable sputtering and minimal damage to the silicon surface.
In our study, we investigated facet formation on silicon surfaces under Xe ion irradiation, examining various factors such as ion incidence angle, fluence, and temperature. We observed that facets formed most prominently at an ion incidence angle of 80°, with surface roughness peaking under these conditions. The temperature also played a significant role in enhancing facet arrangement.
The unique properties of Xe ions—being heavier than Ar ions—led to deeper penetration and a different energy distribution, which influenced the facet formation compared to lighter ions like Ar. Numerical simulations using the Anisotropic Kuramoto-Sivashinsky (AKS) equation complemented our experiments, showing that the facets evolved dynamically over time, further validating our observations. Overall, Xe ions at 0.5 keV provided the right conditions to explore detailed facet patterns and allowed for a deeper understanding of the underlying mechanisms of surface modification.
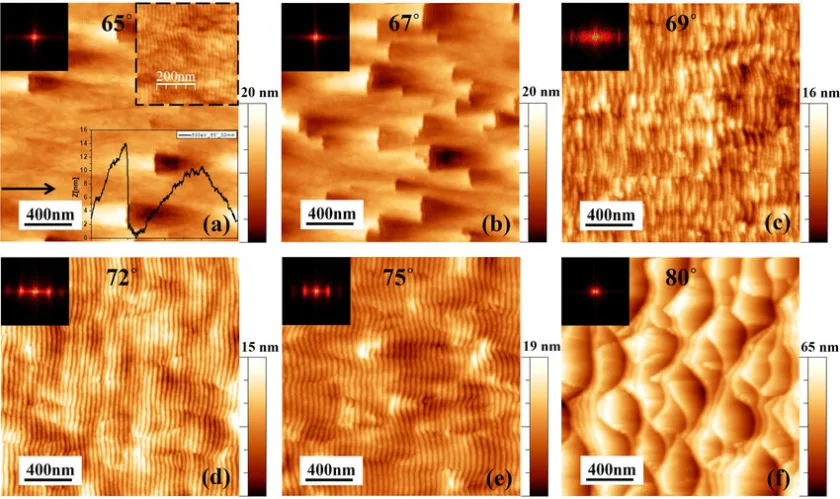
What role does curvature-dependent sputtering yield play in the evolution of these facets? How do dispersion and diffusion interact with this process?
Curvature-dependent sputtering yield plays a crucial role in the evolution of facets on a surface. It refers to how the sputtering rate varies with the surface curvature, which influences the formation and morphology of different facets. This effect is significant because it can alter the local sputtering rates, leading to the development of distinct surface features. In our study, we used numerical simulations to analyze the impact of curvature-dependent sputtering, dispersion, and diffusion on facet evolution.
The simulations were based on a non-linear continuum equation that incorporates these factors. Specifically, the equation of motion (EOM) used for the simulations includes terms for curvature-dependent sputtering, dispersion, and diffusion. The curvature-dependent sputtering term affects how surface features evolve by varying the sputtering rate based on the local curvature. Dispersion influences how particles spread out across the surface, affecting the formation and arrangement of patterns.
In our simulations, dispersion was found to modify the ripple patterns and their velocities. This effect is crucial because it helps to understand how surface features develop and evolve over time. Diffusion involves the movement of atoms across the surface, contributing to the alignment and organization of facets. Our simulations showed that reducing the diffusion coefficient led to better alignment and more regular facet patterns.
This finding aligns with our experimental results, where temperature variations affected facet arrangement. Overall, the interplay between curvature-dependent sputtering yield, dispersion, and diffusion determines the final surface morphology. By incorporating these factors into our simulations, we gained valuable insights into how these processes collectively influence the formation and evolution of facets on the silicon surface under Xe ion irradiation.
In your study, you evaluated the shelf life of plasma-induced hydrophilicity under different storage conditions. Which storage environment best preserved the hydrophilic nature of the treated catheter surfaces?
Water as a storage medium best preserved the hydrophilic nature of the plasma-treated catheter surfaces. This is because water molecules engage in hydrogen bonding with the polar functionalities on the surface, which helps maintain their stability.
Additionally, water restricts the movement of polymeric chains and prevents the diffusion of polar functionalities towards the bulk polymer matrix, thus maintaining the hydrophilic properties over time.
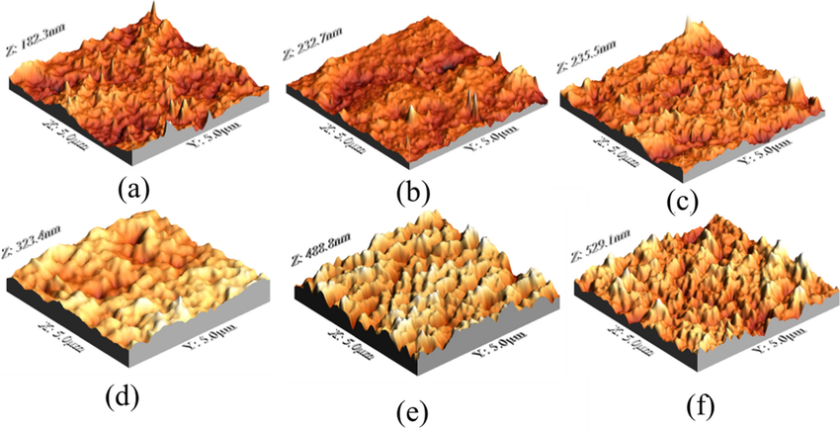
How does your study’s approach compare to other antibacterial surface treatments for catheters? What advantages does the oxygen plasma treatment offer?
Traditional antibacterial surface treatments often involve coatings with antibiotics, antibacterial agents, metals, or metallic nanoparticles. However, antibiotics can lead to bacterial resistance over time, and metallic coatings may be cytotoxic. In contrast, oxygen plasma treatment modifies the catheter surface at the nanoscale without using any toxic materials. This approach not only avoids issues of resistance and cytotoxicity but also enhances the surface’s antibacterial properties in a more sustainable and biocompatible way.
What are your other interests besides academic commitments and research responsibilities… reading, painting, gardening, skiing maybe?
Besides my academic work, I have several interests that keep me engaged and relaxed. I’ve been playing badminton since my master’s studies, and it’s a sport I really enjoy.
Gardening is another passion of mine; it’s the most relaxing activity for me. My father and I created a large garden at home that people often refer to as a greenhouse, and I’ve earned the affectionate nickname “plants mom.” I also love reading, particularly fiction, and watching sci-fi web series, which adds a fun dimension to my leisure time.
Someone comes up to you and says, “I wanna be just like you. I want to be a Plasma Physicist. What advice would you give?”
I’m actually more of a materials scientist than a plasma physicist, but I can share some insights based on my experiences. I would recommend building a strong foundation in mathematics and physics, as these are crucial for understanding complex scientific concepts.
Stay curious and engaged with the latest developments in the field. I also find inspiration in Richard P. Feynman’s seminal lecture ‘There’s Plenty of Room at the Bottom,’ where he envisioned that manipulating matter at the nanometre scale could lead to groundbreaking advancements in science and materials research.
Embrace hands-on experiences through internships or research projects and connect with professionals and academics in your field to gain valuable insights and guidance. This approach can help you navigate and contribute to the exciting world of scientific research.
Quick bits:
What is your favorite movie quote?
“The only limit to our realization of tomorrow is our doubts of today.” – Franklin D. Roosevelt
Your favorite scientific innovation of the 21st century?
My favourite scientific innovation of the 21st century is the development of nanomedicine, particularly in targeted drug delivery systems. These innovations use nanoparticles to deliver medications directly to specific cells or tissues, significantly improving treatment efficacy and minimizing side effects. For instance, advances in nanomedicine have led to more effective treatments for cancer, where nanoparticles can target and destroy tumour cells while sparing healthy tissue.
This targeted approach not only enhances the precision of treatments but also holds the potential to revolutionize how we treat various diseases, offering tremendous benefits to society by improving patient outcomes and quality of life.
What will your TED Talk be 10 years from now?
“The Future of Nanotechnology: Shaping the World at the Atomic Level.”
If you could solve one global issue, what would it be?
Addressing climate change to ensure a sustainable and healthy planet for future generations.
What books should I read in 2024?
Consider “The Midnight Library” by Matt Haig for an intriguing exploration of life choices and regrets, and “The Code Breaker” by Walter Isaacson for an insightful look into the life and work of Jennifer Doudna and the CRISPR technology.
(Wow! Thank you, Dr. Hans, for an incredibly inspiring conversation! Your work is a true source of inspiration. We eagerly anticipate our next visit to witness more of your innovative research. Until then, we extend our best wishes for your continued success in all your future endeavors.)